14:15 |
0066.
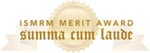 |
Arterial Input Function
for Bolus Tracking Perfusion Imaging in the Brain 
Elias Kellner1, Irina Mader2,
Daniel Nico Splitthoff1, Marco Reisert1,
Katharina Förster3, Thao Nguyen-Thanh2,
Peter Gall1, and Valerij G. Kiselev1
1Department of Radiology, Medical Physics,
University Medical Center Freiburg, D-79106 Freiburg,
Germany, 2Section
of Neuroradiology, Neurocenter of the Freiburg
University Hospital, D-79106 Freiburg, Germany, 3Department
of Cardiovascular Surgery, Albert-Ludwigs-University
Freiburg, D-79106 Freiburg, Germany
Dynamic susceptibility contrast MRI is a well-known
method for determination of perfusion parameters in the
brain. The major challenge of the method is to measure
the contrast inflow into the brain, commonly, the
arterial input function (AIF). The measurement of the
AIF is subject to a number of problems such as signal
void in blood, nonlinear dependence on contrast agent
concentration and partial volume effects. In this study,
those problems are solved with an extension of a
conventional perfusion pulse sequence. Results obtained
in an animal model reproduce known values of the cardiac
output and the cerebral blood volume.
|
14:35 |
0067. |
Nonlinear Formulation of
the Magnetic Field to Source Relationship for Robust
Quantitative Susceptibility Mapping 
Tian Liu1, Cynthia Wisnieff2,3,
Min Lou4, Weiwei Chen5, Pascal
Spincemaille3, and Yi Wang2,3
1MedImageMetric LLC, New York, NY, United
States, 2Biomedical
Engineering, Cornell University, Ithaca, NY, United
States, 3Radiology,
Weill Cornell Medical College, New York, NY, United
States, 4Neurology,
The Second Affiliated Hospital, Zhejiang University
School of Medicine, Hangzhou, Zhejiang, China, 5Radiology,
Tongji Hospital, Tongji Medical College, Huazhong
University of Science& Technology (HUST), Wuhan, Hubei,
China
Quantitative Susceptibility Mapping is becoming an
increasingly active area of scientific and clinical
investigations. In practical applications, there are
sources of errors for QSM including noise, phase
unwrapping failures and signal model inaccuracy. To
improve the robustness of QSM quality, we propose a
nonlinear data fitting for field map estimation and
dipole inversion to reduce noise and phase unwrapping
failures, and a method for model error reduction through
iterative tuning. Compared to the previous linear QSM
method, this nonlinear QSM method reduced checkerboard
pattern in high susceptibility regions in healthy
subjects and markedly reduced artifacts in patients with
intracerebral hemorrhages.
|
14:55 |
0068.
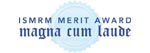 |
Chemical Shift Induced
Phase Errors in Phase Contrast MRI 
Matthew J. Middione1,2, and Daniel B. Ennis1,2
1Department of Radiological Sciences,
Diagnostic Cardiovascular Imaging Section, University of
California, Los Angeles, CA, United States, 2Biomedical
Physics Interdepartmental Program, University of
California, Los Angeles, CA, United States
Phase contrast MRI (PC-MRI) is subject to numerous
sources of error, which decrease both quantitative
accuracy and clinical confidence in the reported
measures. Perivascular fat surrounds most vessels and
can chemically shift across the vessel wall into the
lumen, thereby superposing the complex off-resonant fat
signal onto the complex signal in a pixel containing
flowing blood. This phase error can lead to a clinically
significant over- or underestimation of net forward flow
(10-20mL). A judicious choice of bandwidth and TE can
reduce the chemical shift induced phase errors in PC-MRI
net forward flow measurements to clinically
insignificant levels (<5mL).
|
15:15 |
0069.
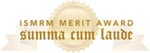 |
Spatially Resolved
Extended Phase Graphs: modeling and design of multi-pulse
sequences with Parallel Transmission 
Shaihan J Malik1, and Joseph V Hajnal1
1Robert Steiner MRI Unit, Imaging Sciences
Department, MRC Clinical Sciences Centre, Hammersmith
Hospital, Imperial College London, London, London,
United Kingdom
RF inhomogeneity correction using parallel transmission
usually focuses on optimising RF field uniformity.
Although improving field homogeneity generally improves
images, most imaging sequences employ rapid successions
of RF pulses, making the received signals depend on the
combined history of many pulses interspersed with
periods of relaxation. Independent modulation of
separate pulses provides additional degrees of freedom
for manipulation of resulting signal. Using a spatially
resolved extended phase graph (SREPG) signal model, RF
inputs may be optimised to produce uniform signals from
non-uniform fields. Improved image homogeneity with more
uniform contrast is demonstrated on 3D TSE brain imaging
at 3T.
|
15:35 |
0070.
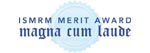 |
In vivo O-Space
imaging with a dedicated 12 cm Z2 insert
coil on a human 3T scanner using phase map calibration 
Jason Stockmann1, Gigi Galiana2,
Leo Tam1, Christoph Juchem2,
Terence Nixon2, and Robert Todd Constable1,2
1Biomedical Engineering, Yale University, New
Haven, CT, United States, 2Diagnostic
Radiology, Yale University, New Haven, CT, United States
We present the first accelerated in vivo "O-Space"
images acquired using a quadratic gradient insert coil
in combination with conventional linear gradients. We
achieve a highly accurate calibration by using phase
encoding to map the spin phase in each voxel at every
readout point of the O-Space pulse sequence, accounting
for effects of field strength, timing, concomitant
fields, and eddy currents. O-Space images compare
favorably to under-sampled radial and Cartesian images
at high acceleration factors using only 8 receive coils.
This work paves the way for more in-depth future
investigation of projection imaging with nonlinear
encoding fields.
|
15:55 |
0071.
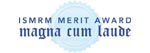 |
A Kinetic Model for
Vessel-Encoded Dynamic Angiography with Arterial Spin
Labeling 
Thomas W Okell1, Michael A Chappell1,2,
Ursula G Schulz3, and Peter Jezzard1
1FMRIB Centre, Nuffield Department of
Clinical Neurosciences, University of Oxford, Oxford,
Oxfordshire, United Kingdom, 2Institute
of Biomedical Engineering, Department of Engineering,
University of Oxford, Oxford, Oxfordshire, United
Kingdom, 3Stroke
Prevention Research Unit, Nuffield Department of
Clinical Neurosciences, University of Oxford, Oxford,
Oxfordshire, United Kingdom
Vessel-encoded dynamic angiography with arterial spin
labeling is able to produce artery-specific images
qualitatively showing the blood flow patterns, vessel
morphology and hemodynamics. Here we develop a kinetic
model to describe the signal in such acquisitions,
allowing the generation of parameter maps relating to
blood volume, arrival time and dispersion, which may
provide useful biomarkers of disease. These parameters
are also used to generate intuitive inflow images and
calculate the relative blood volume flow rates in
downstream vessels from each feeding artery. Results
from application of these methods in healthy volunteers
and a patient with Moyamoya disease are shown.
|
|